In recent days (especially end of September and beginning of October 2023) it became obvious that Russia is using more and more heavy bombs (designated as FAB-500 and FAB-1500) equipped with guidance kits to hit high-value targets from the front lines to deep into the Ukrainian rear. Previously used FAB-250s (which Russia has in the tens of thousands) showed that the concept of gliding munition is very effective in hitting and eliminating valuable targets, and the next logical step was to mount glide & guidance kits to a heavier ordnance. As time passes, more and more of these formidable weapons, once only intended to be dropped from planes in the proximity of the targets, will be launched far from the targets and out of the air defensive cover. They will hit targets with precision that matches and surpasses the best JDAM munition used by NATO.
The dark days for the fortified positions, underground bunkers, warehouses and anything of value are coming to Ukraine.
Lets first talk about the effects of ammunition on the target.
The following are samples from a new book “Rockets and Missiles Over Ukraine”, that will be publish by the end of October 2023 by Pen & Sword Frontline books.
Explosion Effects on Targets
The impact of explosive munitions can be broken down into the principal damage mechanisms and their primary effects, and the secondary and tertiary effects occasioned by these. This section focuses on the primary damage mechanisms and secondary effects of explosives.
The primary effects of explosive weapons are defined as those “caused directly by the destructive effects that radiate from a point of initiation and include blast overpressure, fragmentation, heat and light.” These are attributed directly to the principal damage mechanism of an explosive weapon — blast, fragmentation, and heat. The term “blast” refers to a high-pressure blast wave moving at supersonic speed, referred to as the shock wave, which is followed by blast winds. Primary fragmentation is comprised of fragments that originate directly from the explosive munition. The third damage mechanism is the thermal energy released during detonation of the explosive.
Most high-explosive warheads are not designed to deliver an augmented incendiary effect and the thermal effect is limited to the immediate area of the detonation, primarily because of its extremely short duration. Generally, the primary thermal hazard posed by an explosive weapon is less significant than the blast and fragmentation threats. Secondary effects of explosive weapons derive from the environment in which the munition detonates. The most significant secondary effects include secondary fragmentation, firebrands, ground shock, and cratering.
Secondary fragmentation originates from objects that have been affected by the detonation and can include such objects as pieces of masonry or glass from structures, or bone fragments from human or animal targets. Secondary fragments are generally larger than primary fragments and tend not to travel as fast, or as far.
Ground shock results from the energy imparted to the ground by the shock wave caused by an explosion, and can result from a detonation under or on the ground, or in the air above. Ground shock poses an additional threat to the structural integrity of buildings (which has significant effect in the urban areas), as the ground conducts the shock wave into the foundations and walls.
Cratering
Cratering refers to the buckling and deformation of the ground around the detonation point. Both ground shock and cratering can cause substantial damage to underground shelters and bunkers as well as critical infrastructure. This may be a deliberate effect of explosive munitions optimized for cratering, intended to obstruct avenues of approach or to disrupt infrastructure.
Spalling presents an additional danger in urban environments. It is a stress-wave effect most commonly observed in materials more brittle than metal. This occurs when an impact strikes the outer surface of a solid body, causing fragments to break off from the inside surface. The projectile or the fragment does not need to penetrate the solid body; merely striking the outer surface with sufficient energy may result in spalling. A possible scenario resulting in spalling is a brick wall being struck by a blast wave, or in some cases a projectile or a sufficiently energetic fragment, causing secondary fragmentation inside the building. A significant hazard unique to urban environments is the risk of fatally compromised structural integrity of buildings caused by the blast waves. Any people in and around those buildings and structures may be crushed by their partial or complete collapse.
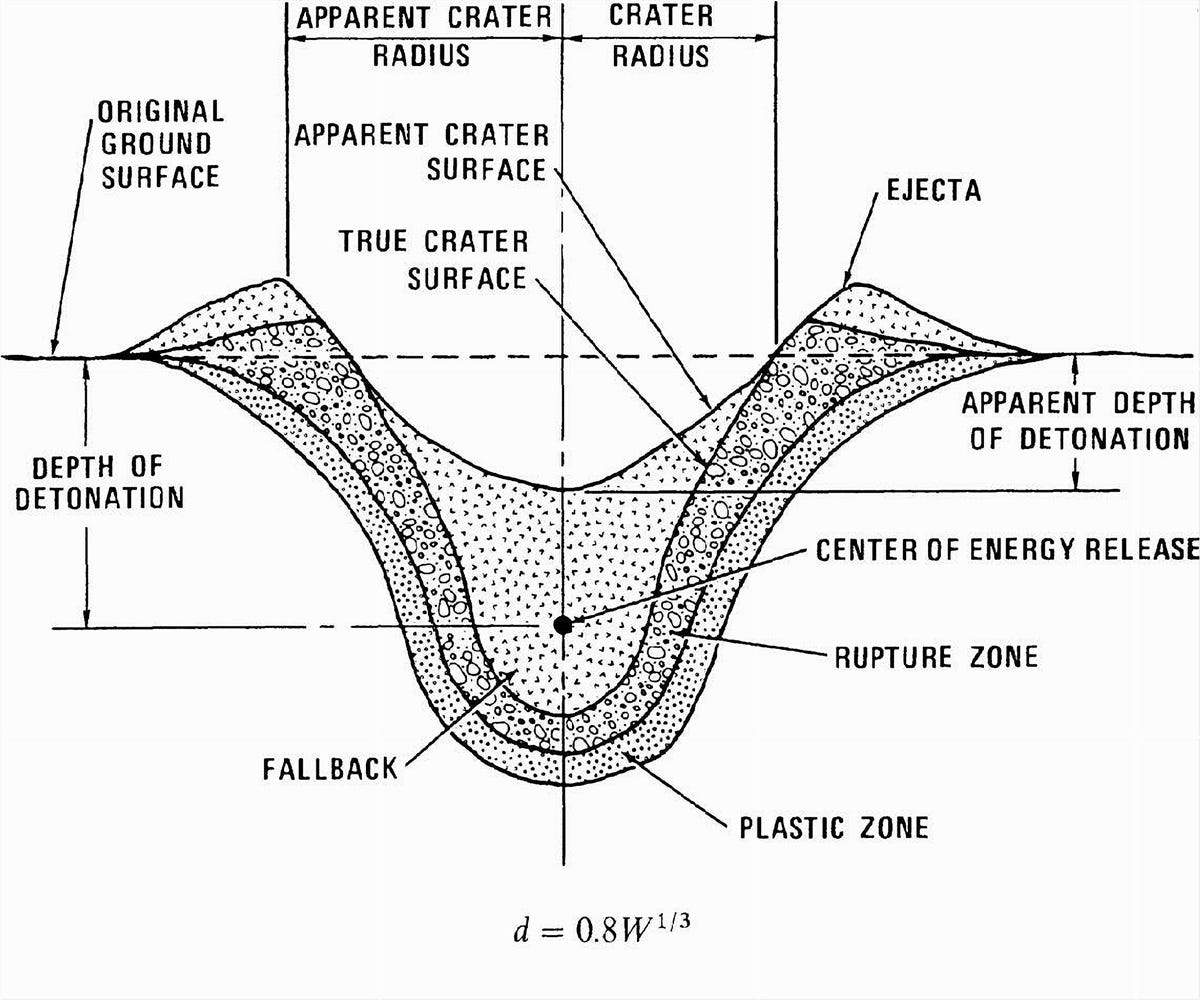
An impressive aspect of a surface explosion is its resulting crater. The great variability in crater formation is indicated by standard deviations of about one-third the diameter given by equation in the picture above.
The depth of the crater created by an explosion is ordinarily about one-quarter its diameter, but this depends on the type of soil present. The diameter of the crater from an explosion also depends on the location of the explosion relative to surface level. Thus, explosions above a surface may not create any crater at all. For explosions below the surface, crater diameter initially increases with depth of explosion, reaches a maximum, then decreases substantially.
There is a comparatively good correlation between crater radius and sympathetic detonation distance, an observation that confirms the thought that sympathetic detonation is affected by the tangible physical means of missile or fragment impact.
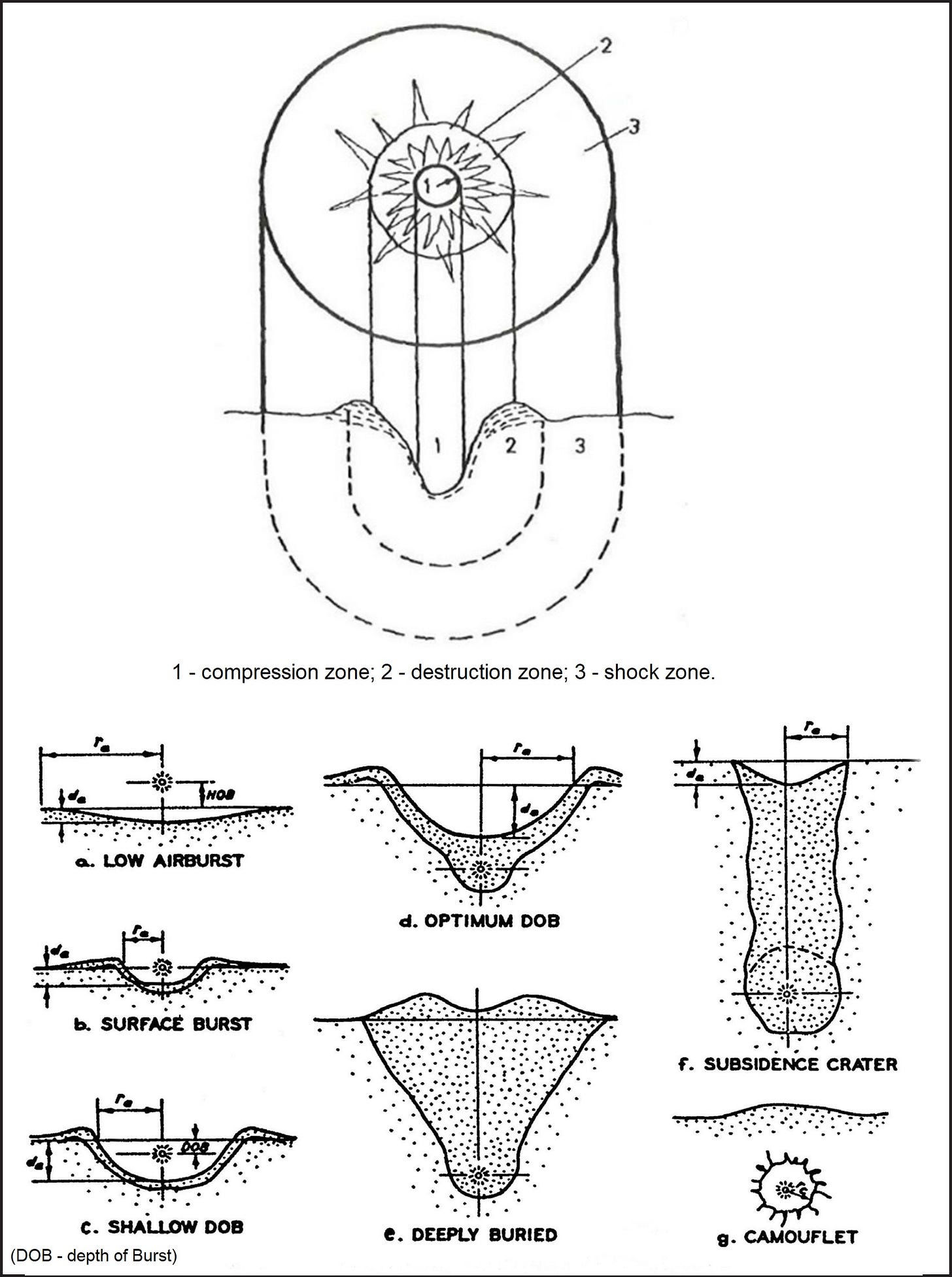
Blast Effects
An explosive is a material that is capable of producing an explosion by releasing the potential energy contained within it. All high explosives produce heat and gas. When a high-explosive charge detonates, it produces a blast wave (overpressure) that consists of two parts: a shock wave and a blast wind. The blast wave pushes outward from the core of the detonation at supersonic speed. The outer edge of the blast wave is made up of the compressed gases contained in the surrounding air. This layer of compressed air is more properly described as a shock wave or shock front. In open air, the blast decays extremely quickly with time and distance; typically it can be measured in milliseconds.
The blast wave has two phases. The positive-pressure phase pushes a large portion of the surrounding air away from the core of the detonation at supersonic speed, leaving a broad partial vacuum behind it. When the blast wave of the positive-pressure phase loses momentum, the partial vacuum behind it causes the compressed and displaced gases to reverse their movement and rush inward to fill the void. The negative-pressure phase moves less quickly than the positive phase and it generally lasts approximately three times as long.
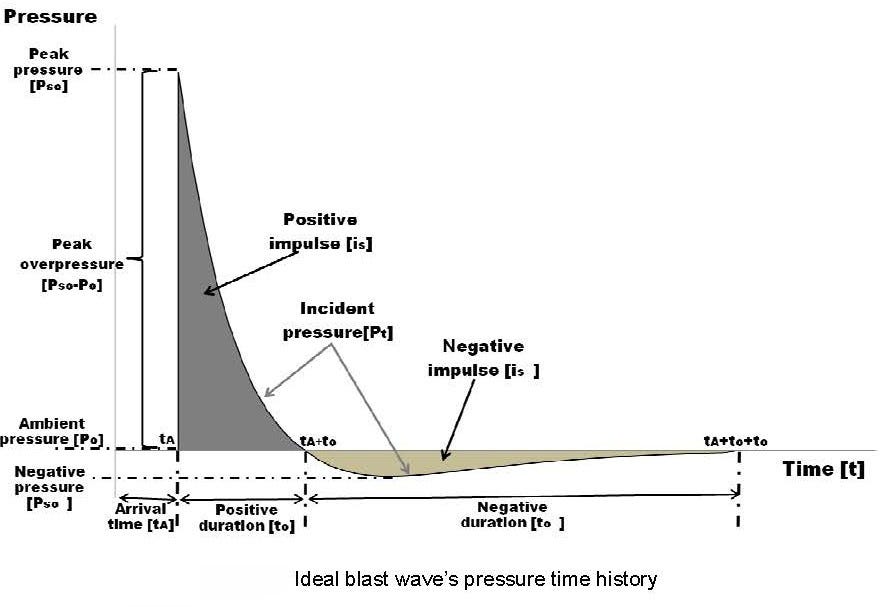
The effect of the pressure wave upon a structure depends on what the structure is composed of and how it is built. In essence, it is dependent upon the structure’s natural frequency of vibration compared with the duration of the blast wave. When the supersonic shock front from a detonation encounters a solid structure, some of the energy is reflected, and some of the energy is transmitted into the structure; the relative amounts depend on the properties of the structure.
In the process of striking the target, the shock front will impart significant momentum to the exterior components. These components will be pushed towards the interior by the positive-pressure wave, straining the resisting elements of the structure (such as support columns, building facades, etc.). Some of those resisting elements, windows in particular, will fail. As the negative-pressure phase of the pressure passes back through the structure, the direction of the energy is reversed. Unlike the reflection of sound waves, which have a negligible effect on the medium through which they are travelling, shock waves are moving at such high speed and contain so much energy that they change the medium itself. When the shock wave hits the ground, it is reflected back into the still-advancing blast wind. This amplifies the blast overpressure anywhere up to twenty times that of the initial detonation.
The main subject of this article is an underground explosion. There are several public studies and works addressing this subject. What is of particular interest for this article is how the bomb affects the underground structure. One of the authoritative books that I can’t recommend enough is the work of professor Igor Balagansky from the Novosibirsk State Technical University in which he and his associates address the damaging effects of the weapons and ammunition. In the Chapter 3 “СРЕДСТВА ПОРАЖЕНИЯ И БОЕПРИПАСЫ ФУГАСНОГО ДЕЙСТВИЯ” he addresses the detonation of a high explosive (in Russian ФУГАС) charge underground. An underground explosion, is one of the main explosion actions in the military use of high-explosive munitions filled with condensed explosives. Some important targets are located at considerable depths to protect them from powerful explosions. Also, an explosion in the ground can create conditions for damage or destruction of above-ground or underground structures.
Unlike air and water, the physical properties (density, strength, elasticity, water saturation, porosity, plasticity, etc.) of soils are extremely diverse. For example, the velocity of sound varies from 100 m/s for peat to 3000 m/s for limestone. The main distinguishing feature of soils is their porosity (i.e. the presence of inclusions in the form of water and trapped air). The volume of such inclusions can vary from 0.1% in granite to 70% in clay. When compressing the porous soil, at first it is compressed due to the elimination of porosity. At the same time, the density of the soil increases significantly. A further increase in pressure leads to compression of the solid particles of the soil.
In addition, the soil, like any solid medium, has residual deformation. After the load is removed, the density of the ground remains almost the same as at the moment of its compression, and the ground particles do not return to their original position. Finally, a considerable part of the explosion energy is spent on irreversible losses associated with the creation of plastic deformations and destruction (crushing, loosening) of soil particles.
The theoretical investigation of the phenomenon of the explosion of a charge in the ground encounters difficulties due to the lack of a general equation of the state for it. The issues determining the destruction of the ground under explosive loads have been insufficiently investigated. Besides, when naturally occurring, soil and rocks are usually layered media with variable layer thicknesses. The properties of each layer are essentially individual, which makes studying the propagation of wave processes in such media a difficult and uncertain task. The diversity of soils and their physical properties and characteristics makes it necessary to use various simplified models that are valid only for certain types of soils. When solving practical problems, experimental data are widely used.
The less compressible the medium (i.e. the higher the sound velocity in the medium) is, the earlier the shock wave (SW) detachment from the explosion product (EP) occurs. For example, for rocky and water-saturated soils, the SW detaches from the EP almost immediately; for porous soils, the more porous they are, the later the detachment occurs. The initial velocity of propagation of the SW only slightly exceeds the velocity of sound in the ground and already at distances equal to 2–3 specified radii of the charge becomes equal to the velocity of sound. The behavior of the time dependence of the pressure at the shock wave front is largely determined by the plastic properties of soils.
For the underground explosion it was found by experience that the radius of the fracture zone in meters can be estimated by the formula:
where mT is the TNT equivalent of the explosive charge, (kg); kf is the coefficient that depends on the properties of the soil.
Medium kf
Freshly sprinkled soil 1.40
Regular soil 1.07
Dense sand 1.04
Stony soil 0.96
Clay 0.94
Limestone 0.92
Granite 0.77
Concrete 0.77
Reinforced concrete 0.65
For the underground bunkers, the medium of interest is concrete and reinforced concrete.
The radius of the compression zone is proportional to the radius of the fracture zone:
The maximum pressure pm at the front of a spherical shock wave and compression waves in the ground can be represented based on similarity theory and dimensions in the form of:
and go to the effect on the underground explosions (Substack is simply not a place to write scientific works which include plenty of formulas and equations even some of them are used for the sake of clarification).
FAB on the target
When a FAB hit the target located underground, three effects are experienced:
1) explosion for the ejection of soil;
2) destruction of underground structures by the explosion;
3) seismic action of the explosion.
Explosion for Ejection
The most visual effect of the explosion is a material ejection.
As mentioned previously, the depth of the crater h is equal to the explosion depth of the charge. The ratio of the crater radius R to the explosion depth h is called the ejection ratio n. Obviously, the volume of the crater W0 can be expressed in terms of its depth h and the ejection index n. A charge with n > 1 is called a charge of increased ejection; if n = 1, such a charge is called a charge of normal ejection; a charge with n < 1 is called a charge of reduced ejection:
The general formulas linking the mass of the TNT equivalent charge to the sizes of the crater can be determined using the theory of similarity and dimensions, taking into account the strength of the ground and the gravity force.
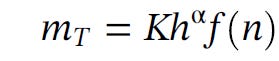
It is necessary to achieve the formation of the craters of the increased ejection, having a large volume. As research shows, the most effective is the ejection ratio n = 1.5–3.0. Sizes of craters are calculated gives an example of experimental data on crater sizes produced during bombing from altitudes of 1200–3500 m by bombs of various calibers with a fuse deceleration of 0.2 second.
Destruction of Underground Structures
In this case, as a rule, a greater depth of penetration is required. Therefore, the use of charges of reduced ejection (n = 0.75–1.00) is advantageous. The destruction of underground objects occurs when the object is within the fracture zone rf = kf 3 mT . The method of equivalent layers is used to calculate the damaging effect. The material layer of the object of destruction with thickness b is replaced by an equivalent ground layer in terms of resistivity be be :
Bomb type Soil Crater Width (m) Crater Depth (m)
FAB-100 Limestone 1.5–2.0 0.7–1.0
FAB-250 Limestone 4.5–5.5 1.2–1.5
FAB-500 Limestone 6.0–8.0 1.5–2.0
FAB-500 Loam 11.0–12.0 4.1–4.5
The mass of the explosive required to fracture an object at a distance of rf is calculated as follows:
Seismic effect
What is also important during the hit of the underground structure is the seismic effect that the explosion will cause.
After the shock wave reaches the surface of the ground, surface waves begin to propagate from the epicenter of the explosion. The reason for the appearance of surface waves is the mass velocity of the soil particles behind the shock wave front. After the SW passage, the soil particles, moving by inertia, pass their equilibrium position and return to their original position.
As a result, there are longitudinal oscillations of soil particles along the direction of the SW movement. At the same time, in the directions perpendicular to the SW movement, transverse oscillations of soil particles arise, connected with the resistance of solid bodies to change their shape.
The joint action of longitudinal and transverse vibrations of soil particles causes a surface seismic wave (Rayleigh wave) to be propagated along the free surface of the ground from the explosion epicenter. The point is that soil particles located on the ground surface experience less resistance from the surroundings during their movement than particles located at depth because in the first case the contact area of particles with the ground is almost twice as small.
The seismic wave causes oscillations of soil particles outside the fracture radius, i.e. in the elastic deformation zone. Recording the horizontal displacements of soil particles under the action of seismic waves makes it possible to obtain the so-called explosion seismogram. In the beginning, the recorder will record the forced oscillations of the soil under the action of the SW. The period of these oscillations corresponds to the elastic deformations of the soil at the shock front (τ = 0.005–0.050 seconds). This phase of oscillations is called onset. After 1–10 seconds after the beginning of the entry phase, the main phase of free soil oscillations, which is a seismic wave, begins.
Conclusion
It is worth saying that the large majority of underground structures were built during Soviet times. Their locations are well known. Ukrainian side can use them as full occupancy or sporadically. In any case, they are potential high value targets especially if some important stuff is there, such as command posts. Some of them are located barely a few meters deep and some are 100+ meters deep. The latter are practically invulnerable to any conventional bomb attack but the use of hypersonic missile with combined warhead and speed even if not penetrating so deep can always cause a seismic effect that can affect the underground structure. When the war is over and the analyses start to kick in it will be known how many of these structures were hit and destroyed but for now only best guessing and rare video clips can give some hints.
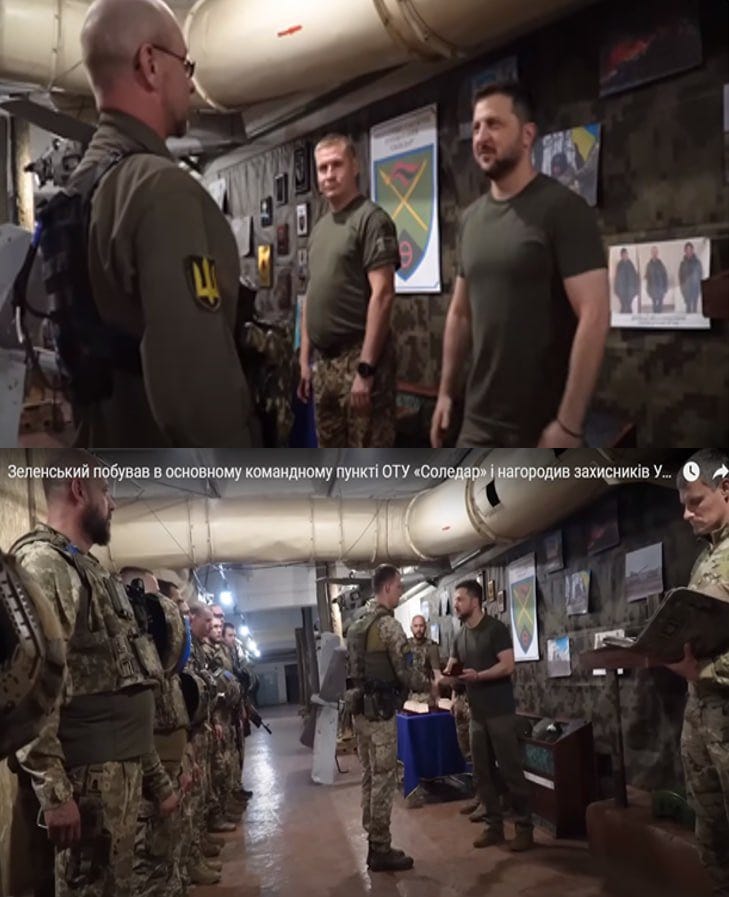
Being underground during the attack is a horrifying experience. the noise is extremely loud, dust all over, shaking and tremors at it best when the structure is not penetrated. Destruction of underground structures can be compared with the destruction of a submarine simply because hardly anyone survives to tell the story.
References:
Rockets and Missiles Over Ukraine, Frontline books
И. А. БАЛА ГАНСКИЙ: ДЕЙСТВИЕ СРЕДСТВ ПОРАЖЕНИЯ И БОЕПРИПАСОВ, НОВОСИБИРСК
I.V. Balagansky: Damaging Effects of Weapons and Ammunition, Willey
G.F Kinney and K.G. Graham, Explosives Shock in Air
S. Jaramaz, Physics of Explosion
Cratering by Explosion, Compendium
[i] Edited by Piquet (EditPiquet@gmail.com)
If you like the article (and much more articles regarding military subjects will come) you can buy me a coffee:
https://www.buymeacoffee.com/mmihajloviW
Thank you
Fascinating stuff--and you kept it simple by using 'speed of sound in a medium' as opposed to 'celerity', a word I've never seen used since my Engineering days.